Drones Deliver Data on Oyster Reef Health
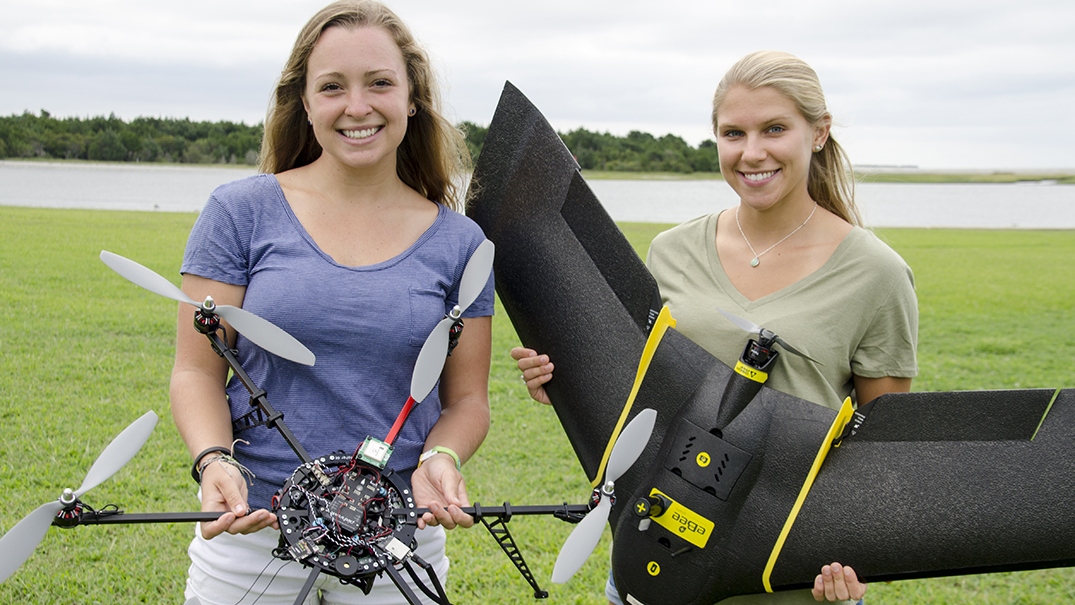
Using aerial imagery from unoccupied aircraft systems, researchers can efficiently assess the condition of oyster reefs.
Guest post by Anna Windle and Sarah Poulin
Oyster reefs play an essential role in coastal and estuarine environments. They provide a suite of ecosystem services, such as providing habitat for juvenile fish, serving as buffers to storm and wave energy, filtering pollutants from the water, and much more.
They’re also pretty tasty. Oysters have been harvested for consumption for centuries. However, today water people in North Carolina only bring in 15 to 20 percent of what was once harvested annually.
How come? Since the early 1900s, North Carolina’s oyster population has declined by an estimated 50-90 percent due to overharvesting, disease, poor water quality and habitat loss, according to N.C. Coastal Federation. Vast stretches of coast-hugging, or fringing, oyster reefs have disappeared, leaving fishermen, conservationists and others concerned about the sustainability of their populations.
In response to the decline, in 2001 the N.C. Division of Marine Fisheries (DMF) implemented management plans and restrictions designed to limit overharvesting. The agency based these new plans and restrictions on the results of a decades-long mapping project. In 1988, DMF began using GPS and geographical information systems, or GIS, to delineate and map habitat types in every estuarine ecosystem in the state.
One of the goals was to determine area and abundance of shellfish, including oysters. Thirty years later, the agency has mapped 90 percent of bottom-dwelling shellfish in those estuarine ecosystems.
Now that baseline mapping efforts of these estuarine ecosystems has been achieved, a quicker and more efficient method is critical to continue monitoring of important shellfish species, such as oysters. A lot can change in three decades, including oyster health, abundance, and the area they cover.
Windle and classmates measure oysters by hand. Windle and Poulin mapped oyster beds like this one in the Rachel Carson Reserve.
In recent years, managers have used aerial imagery from satellites and occupied aircraft to monitor oyster reefs over time. One advantage of these remote-sensing techniques is that they don’t disturb the landscape. However, low-resolution images from sensors on occupied aircraft and satellites can leave out smaller-scale patches of oysters and can inaccurately classify similar-looking habitat types. These methods also can be costly and time-sensitive, and they’re reliant on clear weather.
Unoccupied aircraft systems (UAS), or drones, are transforming the way we collect scientific data. They bridge the gap between sampling in the field and established remote-sensing approaches. Drones can collect significantly higher-resolution imagery, enabling us to see fine details, such as small oyster reef patches.
With increasing battery life and advancements on platforms and sensors, we can now collect thousands of centimeter-scale resolution images of an environment during a 45-minute drone flight across close to 3,000 acres. While established methods can measure to the nearest meter of an oyster reef, drone imagery has the ability to measure individual oysters to the nearest millimeter.
For our project, funded by a North Carolina Sea Grant – NC Space Grant joint fellowship, we used drones to collect data on oyster reefs located in the Rachel Carson Estuarine Research Reserve in Beaufort, North Carolina. We explored different types of drone platforms, sensors and processing options to determine which were the most effective for oyster reef monitoring.
We studied intertidal fringing reefs located on the east end of Town Marsh, an island portion of the reserve, as well as manmade intertidal reefs in Middle Marsh, an area of interconnected marshland located on the west side of the reserve.
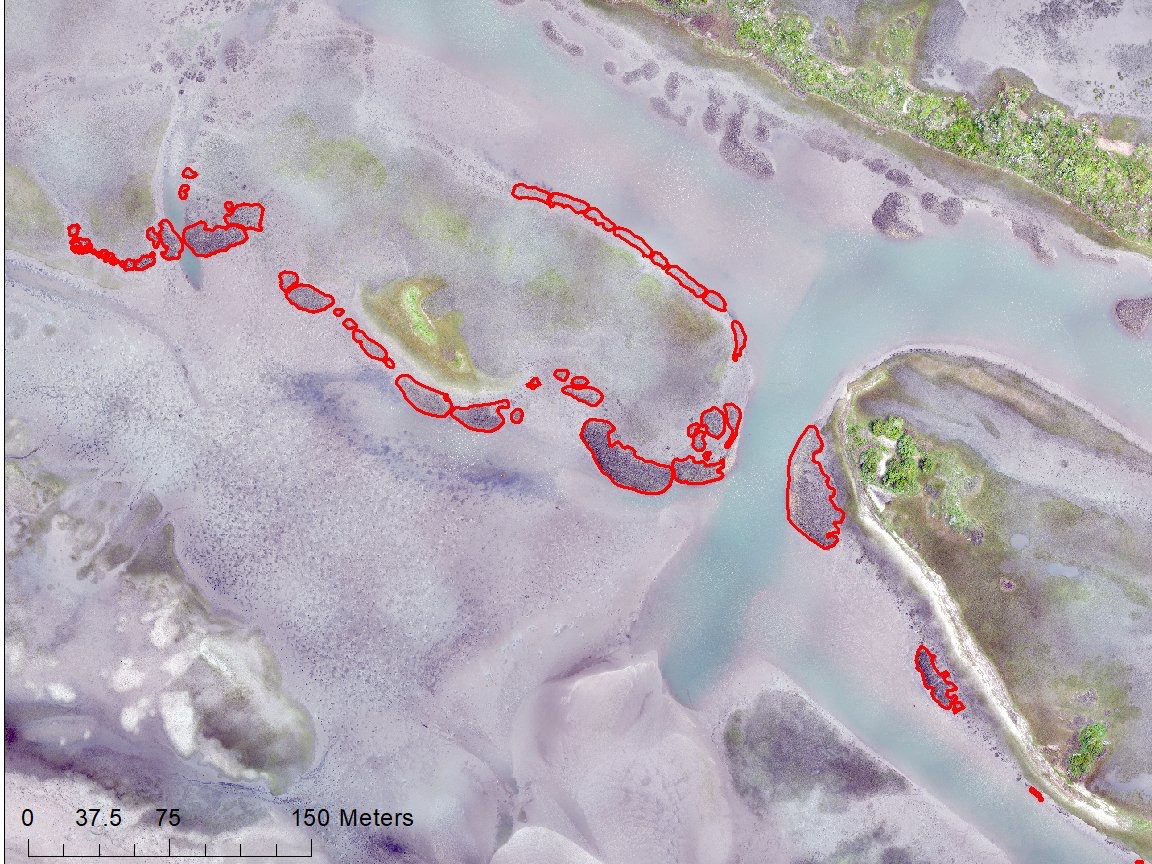
All of the aircraft provided hundreds of high-resolution images. When we got back to the lab, we used a processing software that stitches the images together to form a highly accurate photo representation of an area, known as an orthomosaic. The orthomosaic was geometrically corrected and aligned to a known coordinate system.
Using elevation measurements, the software can also create digital surface models — maps that represent the earth’s surface, including all objects on it. These maps allowed us to analyze the entire area we surveyed.
Reef area and height are widely used metrics for oyster reef monitoring. Using the high-resolution orthomosaic, we delineated the edges of oyster reef patches in order to calculate total oyster reef area. Using the digital surface model, we calculated oyster reef height. We calculated these metrics for a one-square-mile area in just a matter of minutes.
Brandon Puckett, research coordinator for the N.C. Coastal Reserve, had informed us that managers at the Rachel Carson Reserve did not have an accurate estimate of oyster reef habitat in its total 2,315 acres. In a couple days, we were able to identify 11.83 total acres of intertidal oyster reef from analyzing drone imagery of the entire reserve.
Drones Used in This Project
Multirotor drones have multiple propellers allowing the pilot to control the pitch, roll and yaw of the aircraft. This maneuverability allows the pilot to hover over a particular spot, lower the altitude to obtain higher-resolution imagery and launch and recover from a small area.
Fixed wing drones have large wings that provide lift, much like a typical airplane. They tend to have better flight efficiency due to fewer motors and better aerodynamics; however, they require a large take-off and landing area, which can be difficult to find in marine and coastal environments.
Both types of drones can fly manual or autonomous missions. For the majority of our flights, we used a ground control station — in the form of a laptop — to run specific software to plan out the flight pattern in order to achieve optimal sampling. The drones’ autopilot allows it to accurately follow a predetermined path, maintain consistent altitude, and land at a specific location.
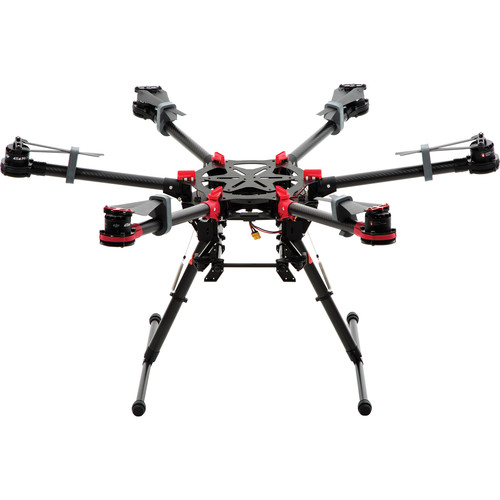
Every drone we used was equipped with a different sensor, or camera. The fixed wing used an RGB sensor optimized for drone applications — the S.O.D.A (Sensor Optimized for Drone Application) camera, which has a large (1.6 cm) 20-megapixel sensor optimized for UAS surveys. We also used a Parrot Sequoia multispectral camera to sample multiple bands of the electromagnetic spectrum (green, red, red edge, near infrared), as well as the senseFly ThermoMAP thermal camera to detect long-wave infrared energy to provide information on temperature. On the multirotor drones, we used standard RGB cameras. The Mavic Pro is equipped with a 1/2.3” CMOS camera and the DJI Matrice 600 used a Sony 6000a digital camera.
We chose to use a combination of aircraft and sensors to determine what was best suited for the area we were surveying in terms of efficiency, image resolution and amount of area covered. We expected the fixed wing to cover more area and fly longer but capture lower-resolution imagery due to the sensor and high altitude. We expected the DJI Matrice 600 hexacopter to capture the best imagery because of the low-altitude flight and high-resolution sensor.
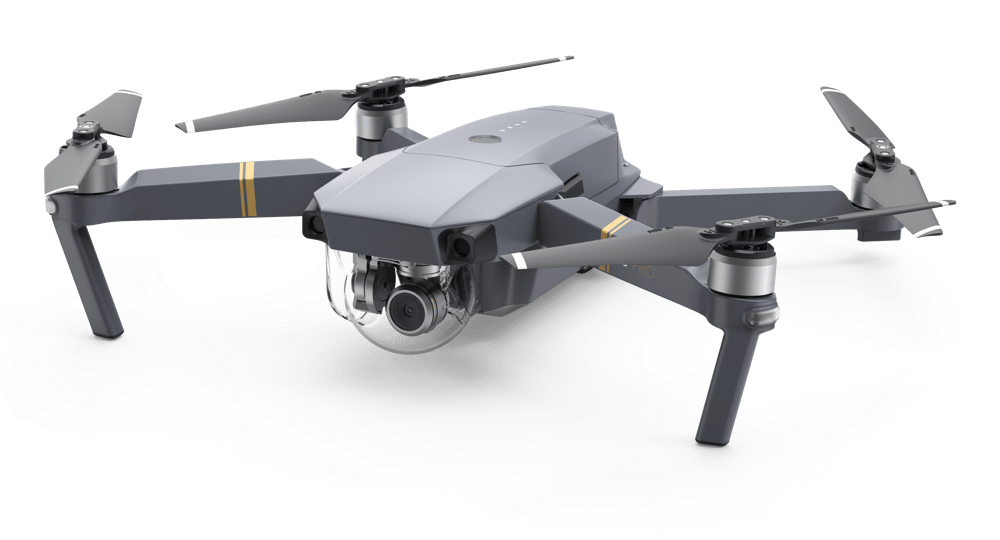
We compared drone imagery of Town Marsh from three different drones: the eBee fixed wing, DJI Mavic Pro quadcopter and the DJI Matrice 600 hexacopter. The eBee flew at an altitude of approximately 57 meters and took a total of 196 images, covering 30.704 acres with one battery. We flew multiple flights over the study area with the DJI Mavic Pro quadcopter using multiple batteries at an altitude of approximately 50 meters. In total, we collected 248 images, covering approximately 2.6 acres. We flew two flights with the DJI Matrice 600 hexacopter at an altitude of 30 meters collecting 687 images over 6.43 acres.
Different drones proved useful for different objectives. Using the fixed wing, we were able to obtain imagery of the entire reserve from 12 flights covering approximately 3,000 acres. Using this imagery, we were able to quantify total acreage of intertidal oyster reef in a matter of days. The DJI hexacopter with the high resolution RGB camera provided 6.13-millimeter resolution imagery. This allowed us to accurately analyze spectral characteristics of oyster reef to learn how to spectrally distinguish them from other habitat classifications.
Some work has been done using different spectral bands to classify and extract reef data. However, these methods are still limited by the spectral similarities of oysters to surrounding substrate or environment (Schill et al., 2006; Le Bris et al., 2016). This research has the potential to produce an automated mapping technique, which would significantly decrease the amount of time and energy necessary to maintain and update pertinent oyster reef maps.
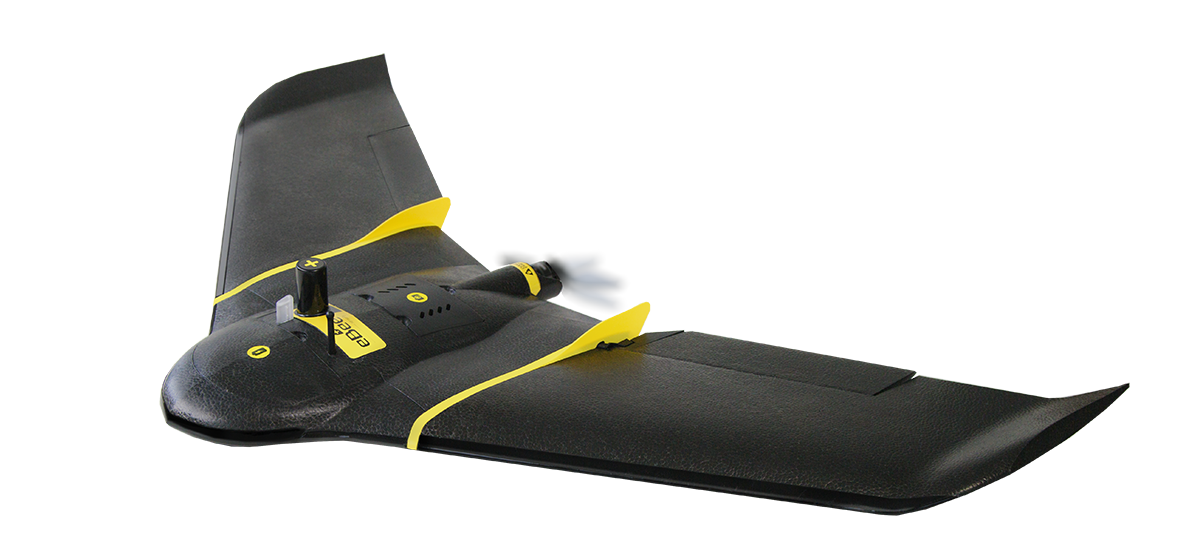
Other important monitoring metrics, such as density and oyster size frequency distribution, have the potential to be analyzed from UAS imagery. Surface rugosity metrics or classified spectral characteristics of a reef can be used to estimate oyster density. This would eliminate the need to excavate and count a sample of live oysters on a reef. With advancing platforms and sensors, UAS imagery could also provide high enough resolution to individually count and measure oysters to estimate the average length and distribution in a reef.
Fixed-wing drones are most likely better for a coastal manager who is interested in mapping large-scale oyster reef habitat, because they can cover larger areas while still capturing small-scale reefs. A multicopter would probably be best for someone interested in the condition of a reef, because it can hover over reefs, fly at lower altitudes and produce high-resolution imagery with information on the density, abundance and potentially the health of the reef.
The drone images form a large mosaic of the coastline. Windle and Poulin used the drone image orthomosaic to more accurately detect oyster beds.
Conclusions
This research will undoubtedly transform the way researchers and managers monitor oyster reef habitat. As our advisor, David W. Johnston of the Duke University Marine Laboratory, noted in a recent article appearing in Annual Reviews of Marine Science, “Drones are poised to revolutionize marine science and conservation, as they provide essentially on-demand remote sensing capabilities at low cost and with reduced human risk.”
Methods involving UAS are quicker, more accurate and less environmentally disturbing than traditional, time-consuming field methods. We hope that the results from our research — which we plan to submit for publication — will help managers and researchers alike to choose the best type of aircraft and sensor for their intended purpose. For more on this project, check out this interactive story map.
After earning master’s degrees in environmental management, Windle and Poulin are continuing to pursue more research opportunities. Windle is now a Ph.D. student at the University of Maryland Center for Environmental Science at Horn Point Laboratory in Cambridge, Maryland, where she uses satellite and UAS remote-sensing methods to assess water quality of the Chesapeake Bay. Poulin is currently a research associate for the Marine Geospatial Ecology Lab at the Duke Marine Lab, with plans to apply to a Ph.D. program next fall.
- Categories: